Ultrasound in Vivo 3D Bioprinting: Harnessing Sound Waves for Life-Changing Therapies
Picture this: with Ultrasound in Vivo 3D Bioprinting, a surgeon waves a slim probe and—without a single incision—a drug-packed micro-capsule or a delicate scaffold of living cells springs into existence inside your body. Sounds like sci-fi, yet a Caltech team has already inched that future forward: they demonstrated that tightly focused ultrasound waves can crosslink specially designed bioinks deep beneath the skin, right where they’re needed.
This breakthrough platform, dubbed “Deep-tissue In Vivo Sound Printing” (DISP), sidesteps the shallow reach of light-based bioprinting and lets clinicians carry out non-invasive additive manufacturing at pinpoint internal sites.
Early animal studies are encouraging: mice and rabbits tolerated the process well, and researchers successfully built structures for targeted drug release, wound-healing matrices, and even proto-bioelectronic implants.
Ultrasound itself isn’t new—radiologists have long used it for imaging, and oncologists for tumor ablation—but this is the first instance of 3-D-printing whole structures inside living tissue. By marrying ultrasound’s deep reach with smart polymer chemistry, the Caltech group unlocked on-demand fabrication of implants and therapies exactly where a patient needs them.
Overcoming Light’s Limits: Why Ultrasound?
Traditional in vivo bioprinting attempts leaned on light (infrared or near-infrared) to kick-start polymerization, yet, as Caltech engineer Wei Gao admits, “infrared penetration is very limited. It only reaches right below the skin.” In short, light falls short whenever surgeons must reach organs more than a few millimetres deep.
Focused ultrasound flips that script. These sound waves can be steered from outside the body and converge to a dot hundreds of times smaller than the beam width, creating a tiny, precisely heated hotspot without harming surrounding tissue. Clinicians already exploit that capability to zap uterine fibroids or bone metastases; the Caltech group simply dialled the energy down to gently warm bioinks by just a few degrees—enough to lock molecules together without incisions.
Recognising ultrasound’s deep-tissue sweet spot, the researchers “turned to a platform that medicine already trusts for penetration and accuracy,” as their news release notes. By letting sound waves carry the where of printing, DISP reaches organs and wounds that lasers can’t, all while keeping the scalpel on the tray.
The DISP Platform: Ultrasound Meets Bioink 🩺
Picture the scene: a clinician inserts a fine needle, releases a smart liquid into damaged tissue, then fires a silent pulse of focused ultrasound. Within seconds, that liquid locks itself into a sturdy, custom-shaped hydrogel—right where it’s needed and nowhere else.
The magic happens inside Caltech’s Deep-tissue In Vivo Sound Printing (DISΠ) platform. First, doctors inject a bioink cocktail—polymer building blocks spiked with therapeutic drugs, living cells, or conductive particles—plus two clever additives:
Low-temperature-sensitive liposomes (LTSLs), loaded with crosslinkers that stay caged at normal body heat.
Gas-vesicle nanobubbles, borrowed from bacteria, that shine brightly on ultrasound images.
Next comes the acoustic wizardry. A steerable transducer nudges the target spot just ~5 °C warmer than its surroundings—enough to make those LTSLs “pop.” Freed crosslinkers immediately snap the monomers together, solidifying the bioink into a tough yet biocompatible hydrogel. Because only the focal point heats up, surgeons can “draw” intricate 3D patterns slice by slice, all without a scalpel.
Meanwhile, the gas vesicles pull double duty. They light up the injected ink on real-time ultrasound and flip their acoustic signal once polymerization starts, giving operators a live confirmation that their structure is forming exactly on cue.
Four pillars make DISΠ tick:
🎯 Focused ultrasound: steered energy that pinpoints deep tissue.
🌡️ Thermosensitive liposomes: tiny heat-triggered “capsules” releasing crosslinkers.
🧬 Polymer bioink: monomers plus optional drugs, cells, or conductive fillers.
🔍 Gas-vesicle contrast agents: on-the-spot imaging & polymerization feedback.
Working in concert, these components deliver on-demand, spatially precise 3D printing inside a living body—an advance many thought was decades away.
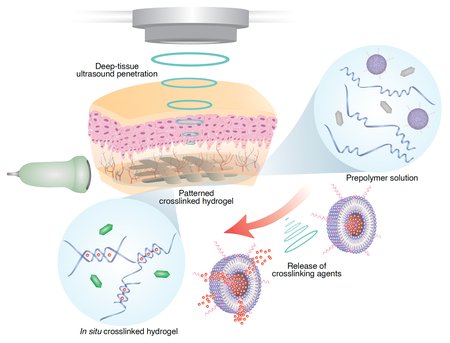
Proof of Concept: Printing Drugs and Scaffolds in Animals 🐭➡️🐇
To prove the idea, Gao’s team injected drug-laden bioink around bladder tumors in mice, then beamed ultrasound to “draw” a chemotherapy depot snug against each cancer. Tumors exposed to the printed gel showed substantially higher cell death over several days than tumors that received the same drug by plain injection.
They didn’t stop there. The researchers printed hydrogels deep inside rabbit muscle and near other diseased tissues, spotting no inflammation or collateral damage afterwards (GenEngNews). Any leftover liquid ink cleared naturally within a week—no cleanup required.
Versatility is where DISΠ really shines. By sprinkling carbon nanotubes or silver flakes into the bioink, the team fabricated electrically conductive hydrogels—potential in-body electrodes that could one day capture heart rhythms from inside the chest. They also printed bioadhesive gels that behave like internal glue, hinting at quick fixes for surgical leaks or trauma wounds.
As Gao points out, “DISΠ lets us print drug-loaded, cell-laden, conductive, and adhesive biomaterials with the same handheld probe.” Looking ahead, the group hopes to seed stem-cell scaffolds inside beating hearts, pairing ultrasound guidance with AI to track moving organs in real time.
Additive manufacturing has officially stepped inside the body—ushering in a toolkit for therapies we once had to dream about.
Technical Breakthroughs: How DISP Differs ⚙️
Several forward-thinking ideas set DISP apart from every other bioprinting approach on the lab bench:
🔊 Ultrasound-Triggered Chemistry — Earlier systems relied on light to spark polymerization, yet DISP flips on the “print” switch with focused ultrasound. Those waves slip deep into tissue, and only at the warmed focal point do low-temperature liposomes crack open to spill their crosslinkers —so the gel sets exactly where the beam lands.
🧪 Smart Bioinks (“US-Inks”) — The injected cocktail is anything but ordinary. It blends monomer precursors (think acrylates or GelMA), gas-vesicle contrast bubbles, therapeutic or cellular payloads, plus the heat-sensitive liposomes that tote crosslinkers. These ultrasound-responsive mixtures—nicknamed “US-inks”—sit quietly until the beam arrives, then snap solid in milliseconds.
🎯 In Situ Precision — Inside the body, DISP follows a digital 3D blueprint just like a desktop printer would. The transducer walks a planned path (or keeps pace with a moving organ under imaging guidance), firing voxel-by-voxel. Gas-vesicle echoes confirm every microscopic gel dot, letting surgeons “draw” intricate shapes beneath living tissue.
🌡️ Minimal Thermal Load — Heating tops out around 5 °C above body temp (≈ 40 °C)—warm enough to melt liposomes but far below the threshold that harms cells. Ultrasound’s long safety record, plus tight control of power and pulse length, keeps cavitation or burns at bay.
🔍 Real-Time Monitoring — Because the ink carries gas vesicles, the whole process is visible. Those bubbles flip their acoustic signature the instant gelation begins, giving operators instant feedback and room to tweak patterns mid-print.
Put together—ultrasound actuation, liposome “heat switches,” next-gen bioinks, and live imaging—DISP forms one seamless toolset that, as GenEngNews notes, “unlocks applications in bioelectronics, drug delivery, tissue engineering, wound sealing, and beyond.”
Potential Medical Applications of Ultrasound in Vivo 3D Bioprinting 🩺
The power to build structures inside the body sparks a whole menu of clinical possibilities:
🎯 Targeted Cancer Therapy — Caltech’s chemotherapeutic hydrogels print snugly around tumors, bathing them in high-dose drug while sparing the rest of the body. Surgeons could even sculpt gels to line cavity walls after tumor resection.
🩹 Wound Healing & Hemostasis — Imagine spraying a bio-adhesive ink and “sewing” an internal patch with nothing but sound—no sutures needed. Post-abdominal surgery, that same trick could seal leaky organs.
💓 Tissue Engineering In Vivo — For heart-attack victims, stem-cell-laden scaffolds might be printed right onto injured myocardium. Orthopedic teams could form bone-mending gels within a fracture gap, all guided by live imaging for patient-specific geometry.
📡 Bioelectronic Interfaces — Conductive hydrogels serve as soft, chronic electrodes: an ultrasound-drawn ECG pad on the heart, a glucose sensor under the skin, or neural probes woven from biocompatible gel circuits.
🧬 Cell & Gene Delivery — By embedding live cells or viral vectors, DISP could plant regenerative “seed beds” or localized gene-therapy depots exactly where they’re needed, improving engraftment and precision.
⛑️ Point-of-Care Implants — Picture paramedics injecting curative hydrogel into a trauma victim roadside, then curing it with a handheld ultrasound wand for instant stabilization.
Bottom line: Any procedure that currently leans on open surgery or whole-body infusions could be rewritten by this non-invasive playbook. Caltech foresees clinicians 3D printing “miniature capsules” of cells or drugs—even inside a beating heart
Focused Ultrasound: A Safe & Seasoned Modality 🔬
One big reason this concept feels doable? Focused ultrasound (FUS) is hardly new—clinicians already use it to ease uterine fibroids, calm bone pain, and tame tremors, and dozens more indications are in the pipeline. Modern FUS rigs can steer acoustic energy deep inside the body under MRI or sonographic guidance, nailing targets with sub-millimetre accuracy.
Decades of lab work have also shown that sound waves can unlock drugs on command: load a chemo or gene therapy into low-temperature-sensitive liposomes (LTSLs), warm the spot to a cosy 40 °C, and—pop—the cargo pours out in high concentration. That’s the very trick the DISP platform borrows: a brief burst of high-intensity focused ultrasound (HIFU) nudges the tissue a few degrees warmer, cracking the liposomes open.
Because FUS is already considered safe when power and dwell time are dialled in, extending it from drug release to polymer printing is less a leap and more a hop. The research team kept their temperature lift mild—barely a five-degree uptick—well within hyperthermia norms, and animal studies showed zero tissue damage. In short, DISP rides on the proven precision of clinical ultrasound, but repurposes those waves as a 3-D paintbrush.
Challenges & Future Directions 🚧
⚡ Precision in Moving Organs – Hearts beat, lungs rise and fall. Engineers are weaving in AI and real-time imaging so the acoustic focus can chase those motions on the fly.
🧪 Bioink Optimisation – So far, only a handful of polymer-liposome recipes gel nicely under ultrasound. Next-gen inks must be bioresorbable, strong enough for load-bearing jobs, and kind to living cells.
🔍 Process–Structure Relationships – Scientists still need to map how frequency, intensity, and pulse length sculpt a hydrogel’s micro-architecture—and then lock those settings for reproducible outcomes.
📑 Regulatory Pathway – Any injectable that hardens in the body invites tough questions about toxicity and long-term clearance. Early data—no inflammation, rapid wash-out of unused ink—looks good, but human trials will demand exhaustive proof.
🚚 Delivery Mechanisms – Today’s protocol relies on a simple needle. Tomorrow’s might thread catheters or endoscopes into harder-to-reach hollows, marrying DISP with existing interventional toys.
Despite the to-do list, excitement is palpable: a recent study from an Israeli institute echoed the promise of sound-mediated polymerisation for everything from cell therapy to in-body 3-D printing—a clear sign this frontier is heating up.
Non-Invasive Manufacturing: A New Medical Blueprint 🏗️
Think of DISP as in-vivo additive manufacturing. Instead of carving a prosthetic on the bench and stitching it in, surgeons could “print” an implant layer-by-layer right where it belongs—no scalpel, no sutures, minimal downtime. Picture building a patient-specific stent, gluing a leaky organ, or weaving a vascular lattice directly inside a beating heart. When the “printer” is an ultrasound beam, the operating theatre dissolves into thin air.
Key Takeaways for Engineers & Researchers 🛠️
Deep-Reach Actuation: Ultrasound sidesteps the depth limits of lasers, placing the voxel wherever the beam can travel.
Smart Bioinks: Polymers + payloads + heat-poppable liposomes form the magic mix.
Live Visuals: Gas-vesicle contrast agents turn the whole show into a real-time hologram.
Therapeutic Wins: Early animal work shows drug depots, glue patches, and conductive gels all print cleanly—with better outcomes and no collateral harm.
Road Ahead: Bigger animal models, broader ink chemistries, AI-guided beam steering, and the long climb toward clinical approval.
Sound-driven surgery is no longer science fiction. By wedding mature ultrasound tech with inventive bioinks, DISP hints at a future where clinicians print cures instead of implanting them—a quieter, gentler era of personalised medicine that might arrive sooner than anyone expected.
References
California Institute of Technology. In vivo 3D printing using sound holds promise for precise drug delivery, wound healing and more.
Science and technology newsCalifornia Institute of Technology. 3D printing in vivo using sound. Caltech.
Research institute newsKtori, S. In vivo bioprinting shows promise for 3D printed implants without surgery. Genetic Engineering & Biotechnology News.
Biotech industry newsBioengineer. (2025, May 16). Ultrasound-guided 3D bioprinting breakthrough allows in vivo deep-tissue implant fabrication. Bioengineer.org.
Biomedical engineering news