3D Printing Infill Patterns to Boost Your Production Efficiency
Thanks to 3D printing, production processes have become more controllable. 3D printing, also known as additive manufacturing technology, offers an advantageous alternative to traditional manufacturing methods like subtractive manufacturing (e.g., CNC). In additive manufacturing, many parameters can be adjusted during the preparation process for printing. Two of the most important parameters are printing density and printing pattern.
When deciding on the density and pattern, criteria such as the following are evaluated:
- The purpose of the object
- Mechanical requirements
- Printing time and cost
Now, let’s take a closer look at 3D printing density and printing patterns.
Density
As we will see in the scientific tests below, infill density directly affects an object’s weight, strength, flexibility, and print time. However, adjusting the infill percentage alone is not sufficient to optimize these parameters for specific applications. To make informed decisions, it is also crucial to understand infill patterns and their impact on the final print.
Infill Patterns
The pattern used to fill the interior of an object during printing is called the infill pattern. Choosing the right infill pattern is crucial for producing a functional final product, as different infill patterns offer specific advantages and disadvantages depending on the object’s intended use and purpose. These patterns directly affect:
- The weight of the object
- Its durability
- Flexibility
- Material consumption
- Printing speed
During the slicing process, we can select infill patterns, and most slicing software (such as PrusaSlicer, Ultimaker Cura, Slic3r, Simplify3D, etc.) allows users to choose from a variety of infill patterns. Below, you can explore objects sliced with different infill patterns using Ultimaker Cura, which offers a wide range of options. Some of these infill patterns include:
Grid, Lines, Triangles, Tri-Hexagon, Cubic, Cubic Subdivision, Octet, Quarter Cubic, Concentric, Zig Zag, Cross, Cross 3D, Gyroid and Lighting.
References
Pernet, B., Nagel, J. K., & Zhang, H. (2022). Compressive strength assessment of 3D printing infill patterns. Procedia CIRP, 105, 682–687.
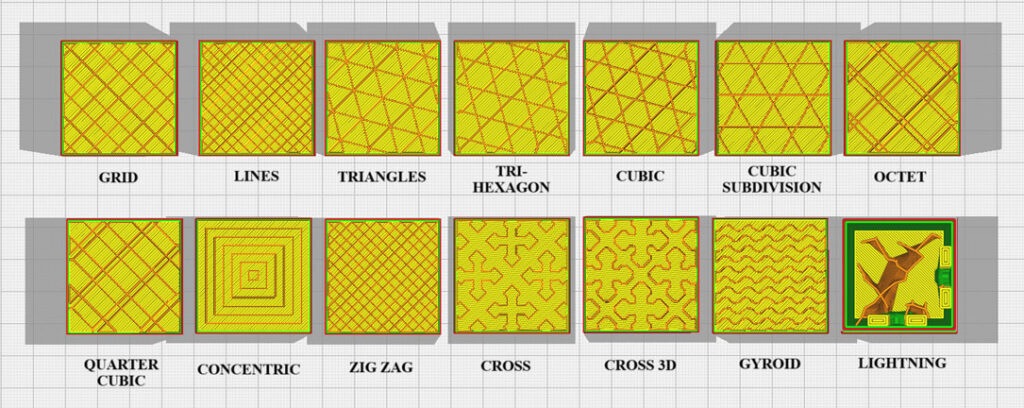
How Can We Choose the Right Pattern?
Is there any scientific research on this topic? Let’s examine the scientific tests and results that help determine which pattern to choose and when.
The study titled “Compressive Strength Assessment of 3D Printing Infill Patterns” presented at the 29th CIRP Life Cycle Engineering Conference successfully evaluates the mechanical strength of various infill patterns at different densities and reveals their behavior under load.
The study involved testing objects with 14 different patterns at 5 different density levels: 20%, 40%, 60%, 80%, and 100%, resulting in a total of 70 tests across all combinations of patterns and densities.
The 14 infill patterns used in the tests were: Grid, Lines, Triangle, Cubic, Tetrahedral, Concentric, Concentric 3D, Zigzag, Gyroid, Octet, Cross, Cross 3D, Tri-hexagonal, and Quarter Cubic.
Below, you can see how these patterns appear when sliced using Slicer software.
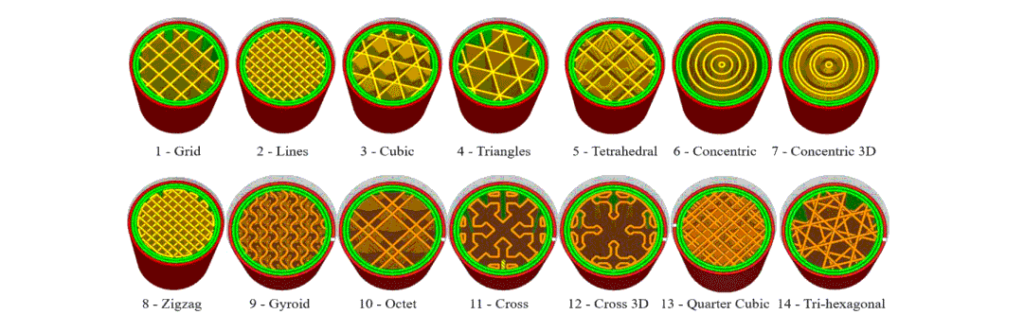
Before moving on to the experiments, it is useful to discuss the conditions under which they were conducted.
The 3D models were created using SolidWorks software. The slicing process was performed with Slicer and Ultimaker Cura software. The objects used in the experiments were printed using the Dremel Digilab 3D45 3D printer.
PLA (Polylactic Acid) was used as the printing material in the experiments, and the printing parameters and experimental setup are as follows.
PARAMETERS | VALUES |
---|---|
Printing Temperature | 230 °C |
Build Plate Temperature | 60 °C |
Layer Height | 0.1 mm |
Wall Thickness | 0.8 mm |
Printing Speed | 50 mm/s |
Reliability and Consistency of the Experiment
Before discussing the test results, it is essential to address the consistency of the procedure. To evaluate the stability and reliability of the experimental setup and testing process, six identical cylinders with a 40% infill density were printed and tested simultaneously.
Among these, the two cylinders that withstood the highest loads showed a difference of only 3% in their load-bearing capacity. This minimal variation demonstrates the consistency and reliability of the test results.
Compression Tests
The goal of the compression tests is to identify the most efficient infill patterns that offer the best performance under maximum load while remaining as lightweight as possible.
The study presents results based on two key metrics:
- Defined Load Weight Ratio (α)
- Maximum Load vs Weight Relationship
Defined Load Weight Ratio (α)
The defined load weight ratio (α) is a metric obtained by dividing the maximum load by the weight of the printed object. This parameter is used to determine which infill pattern provides the best strength-to-weight ratio across different designs.
It is calculated as:
α = Maximum Load / Weight
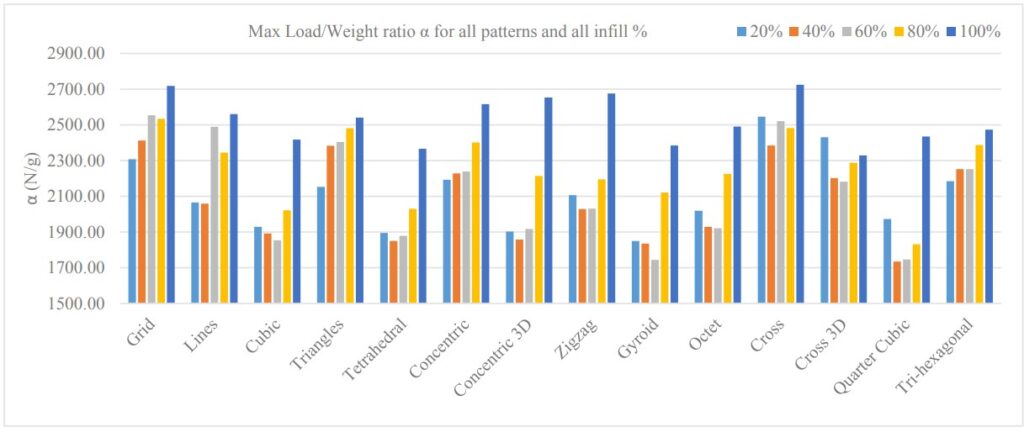
In the graph above, each bar represents a different infill pattern. The results obtained from this graph clearly demonstrate that infill patterns have a direct impact on the mechanical properties of the printed object.
Based on the findings from this graph, we can conclude the following:
- The ratio at 100% infill density is higher compared to the 20% to 60% infill densities.
- Similarly, the ratio at 80% infill density is higher than that of 20% to 60% infill densities, but this trend is decreasing.
- However, at 60% infill density, there is no clear correlation indicating that the ratio would be higher compared to 20% to 40% infill densities.
There are also exceptions to these general trends. For instance, the Grid pattern with 60% infill density exhibits a higher ratio than the Cubic pattern with 100% infill density.
Now, let’s examine the ranking of the tested infill patterns based on their α values in the table below and interpret the results.
Infill Pattern | Average α (N/g) |
---|---|
Cross | 2531.92 |
Grid | 2505.04 |
Triangles | 2392.60 |
Concentric | 2335.80 |
Tri-hexagonal | 2310.20 |
Lines | 2303.82 |
Cross 3D | 2286.24 |
Zigzag | 2207.22 |
Octet | 2117.22 |
Concentric 3D | 2108.83 |
Cubic | 2022.91 |
Tetrahedral | 2004.01 |
Gyroid | 1986.82 |
Quarter Cubic | 1943.71 |
General Conclusion
In general, it can be concluded that 2D infill patterns tend to perform better compared to 3D infill patterns. This is mainly because in 2D infill patterns, the material is consistently placed in the same direction, allowing these structures to manage stress from a single direction more effectively compared to objects printed with 3D infill patterns.
The best-performing 2D infill patterns in terms of load-to-weight ratio are:
- Cross
- Grid
- Triangles
- Concentric
- Tri-hexagonal
- Lines
Among the 3D infill patterns, Cross 3D performed better than Zigzag, demonstrating superior strength characteristics.
Maximum Load-Weight Relationship
The maximum load-weight relationship directly represents the maximum load that a specimen can withstand relative to its weight.
The test results presenting this maximum load-weight relationship are given in the graph below. Here, each shape represents an infill density, while each color corresponds to a specific infill pattern.
Grid (Red), Lines (Dark Red), Cubic (Orange), Triangle (Light Green), Tetrahedral (Green), Concentric (Light Blue), Concentric 3D (Blue), Zigzag (Dark Blue), Gyroid (Gold), Octet (Grey), Cross (Purple), Cross 3D (White), Tri-hexagonal (Yellow), Quarter Cubic (Pink)
This graph provides valuable insights into how different infill patterns and densities affect load-bearing capacity, aiding in the selection of optimal infill structures for specific mechanical requirements.
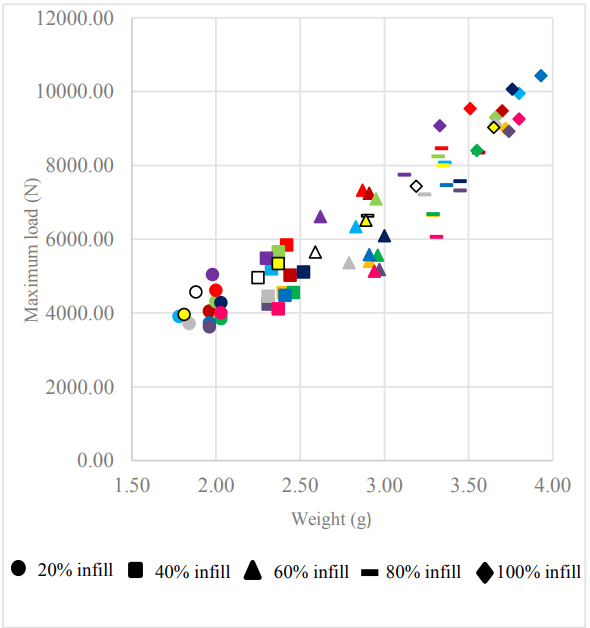
General Observations from the Experiment:
- The test results indicate that as the weight of objects produced with different infill patterns increases, the maximum load they can tolerate also increases.
- The Grid (Red) infill pattern at 40% infill density produced an object weighing 2.42g, capable of withstanding 6000N, making it lighter than other patterns while maintaining the same load capacity. Similarly, a Zigzag (Dark Blue) infill pattern at 60% density provided a comparable load capacity at 3g weight, and a Quarter Cubic infill pattern at 80% density showed similar performance at 3.31g weight, withstanding approximately 6000N.
- 100% infill density does not always provide the highest strength.
- While 3D infill patterns offer more balanced strength distribution in all directions, 2D infill patterns demonstrate superior load-bearing performance.
Conclusion and Comparison
The compression test results show that 2D infill patterns tend to perform better than 3D infill patterns when it comes to strength-to-weight ratio and maximum load capacity.
Patterns like Grid, Cross, and Triangles stood out as the best-performing 2D infill patterns, offering a great balance of lightweight design and durability. This means they’re a solid choice for efficient and strong 3D-printed parts.
Now, let’s take a closer look at each pattern and what these findings mean for them.
Grid
As seen in the test results, this pattern provides higher mechanical strength at a lower density and weight ratio compared to other patterns. It performs particularly well in 40%-80% infill densities, offering better load-bearing capacity. However, it is important to note that this strength is mainly effective against forces coming from a single direction.
This pattern is suitable for lightweight applications requiring strength. It can be used in automotive and aerospace applications, including engine covers, internal structural supports, and fastening elements. Additionally, it can be advantageous in prototyping and sports equipment.
Lines
While it may not be as strong as the Grid pattern, it still offers good mechanical strength relative to its lightweight structure. Since it belongs to the 2D infill family, it has the advantage of fast printing, as nozzle movements are simpler during printing. It provides moderate mechanical strength.
This pattern is ideal for applications where extreme strength is not critical, but lightweight and cost-efficiency are important. It can be used in prototyping applications and artistic designs that do not require high mechanical durability.
Cubic
As seen in the previous tests, the defined load-to-weight ratio (α) is relatively low against unidirectional forces. However, it provides good resistance against multidirectional, 3D forces. Compared to other patterns, it has a slower print speed and moderate material consumption.
This infill pattern is suitable for mechanical parts exposed to forces from multiple directions. It can be beneficial for robotic arms and joints. Additionally, its complex 3D structure makes it a good choice for optimizing heat distribution and airflow management.
Triangles
Ranked right after Cross and Grid in the compression tests, this pattern forms regular triangular structures throughout the layers, making it highly effective in terms of strength-to-weight ratio. Due to these properties, it is widely used in engineering, design projects, and construction applications.
Tetrahedral
As a 3D infill pattern, this structure is suitable for parts exposed to forces from multiple directions. It fills the object using a tetrahedron (four-faced) geometry, providing a stable and precise design solution. This pattern can be beneficial for high-precision and stable structures.
Concentric
Concentric 3D
As observed in the compression tests, this pattern does not perform well under unidirectional forces, but it offers better resistance to multidirectional forces. In addition to its ability to optimize heat distribution, it is also used for aesthetic applications.
Zigzag
Gyroid
Although it does not exhibit high strength against unidirectional forces, it performs well under multidirectional forces. Its aesthetic and complex structure, along with its low material requirements, make it an attractive choice for certain applications. Like the Concentric 3D pattern, it can optimize heat distribution. In summary, this pattern is suitable for applications that require lightweight structures with isotropic strength.
Octet
The Octet pattern fills objects with interconnected octahedral structures, providing excellent multidirectional load resistance. It is suitable for applications in heat and fluid management. Additionally, it is ideal for lightweight enclosures that require good resistance against mechanical impacts from multiple directions. However, its complex 3D structure can extend printing times.
Cross
This pattern ranks first in the compression tests with a defined load-to-weight ratio (α) of 2531.92 N, making it highly advantageous due to its high strength, short printing time, and lightweight characteristics. It uses intersecting lines to build the desired object. It is commonly used in prototype production, medical devices, automotive, and aerospace applications.
Cross 3D
Due to its 3D cross-shaped structure, it provides isotropic strength both internally and externally. While it offers aesthetic and functional benefits, it is also effective for producing lightweight parts. However, as shown in the compression test results, its unidirectional strength is relatively weak, which is a disadvantage.
Quarter Cubic
This pattern utilizes tetrahedrons and their truncated versions to create the printed structure. As a 3D infill pattern, its mechanical strength is low, making it unsuitable for applications subjected to significant loads. Another disadvantage is its long bridging distances, which can negatively affect surface quality.
Tri-Hexagonal
As a 2D infill pattern, the tri-hexagonal structure combines hexagons and triangles to form a unique layout. As observed in the compression tests, its compression strength is at a good level. However, it performs poorly in multidirectional strength. The hexagonal geometry provides an aesthetic and orderly appearance, but the gaps between hexagons can be a disadvantage in certain applications.