Multi-Metal 3D Printing: Revolutionizing Metal Additive Manufacturing
Multi-Metal 3D Printing is transforming how engineers design and build metal parts. We’re genuinely excited 😃 by the idea of blending several alloys inside one printed component, because it opens the door to performance gains—and cost savings—that yesterday’s factories could only day-dream about. This approach—still known by its mouth-full formal name, Multi-Metal 3D Printing or Multi-Material Laser Powder Bed Fusion (MM-LPBF)—lets multiple alloys coexist in a single build, producing intricate shapes with property gradients that plain-old machining or single-alloy AM can’t deliver.
Put simply, multi-metal printing sandwiches the brute strength of one alloy with, say, the corrosion toughness of another, right inside the same part. No wonder many observers hail this branch of metal additive as the next manufacturing revolution: short lead times, huge design freedom, and features like razor-fine lattices or smoothly graded interiors are suddenly on the menu.
What Is Multi-Metal 3D Printing?
Multi-Metal 3D Printing is any additive process that welds two (or more) distinct metal powders into one cohesive build. Unlike single-alloy jobs, multi-metal AM drops each alloy exactly where its properties are needed. A Penn State team recently showcased this idea by printing a complex gyroid out of stainless steel and bronze (90 % Cu, 10 % Sn), proving even delicate lattices can be fused seamlessly from two metals. In practice, engineers can grade a part so one zone is rock-hard and another is heat-conductive or bone-friendly. Imagine a spinal implant whose porous lattice is a ductile, bio-safe alloy while its solid core is ultra-strong. Tailor-made, inside and out.
🎯 Selective Powder Deposition (SPD): This flagship MM-LPBF method relies on a multi-hopper recoater—think an Aerosint drum—to sprinkle micro-patches of different powders side by side in every layer.
🔥 Laser Fusion: Once those powders are down, one or several lasers sweep across the bed, melting each micro-patch. The machine tunes energy per alloy, so every metal fuses cleanly without scorching its neighbor.
🕵️ In-situ Monitoring: High-res cameras and computed-tomography scanners keep an eye on the melt pool, flagging pores, cracks, or dodgy interfaces in real time—so defects get zapped before they grow.
Together, these steps give engineers near-pixel control of material placement, letting them quite literally “paint” with metals at the micron scale. Multi-Material Laser Powder Bed Fusion (MM-LPBF) is the tech leading that charge, snapping onto mainstream AM platforms (3D Systems, EOS, and friends) through bolt-on recoaters and feeders. In real builds, a one-centimeter-tall sample can stack thousands of mixed-metal layers in just a few hours—each one laid down with micrometer precision.
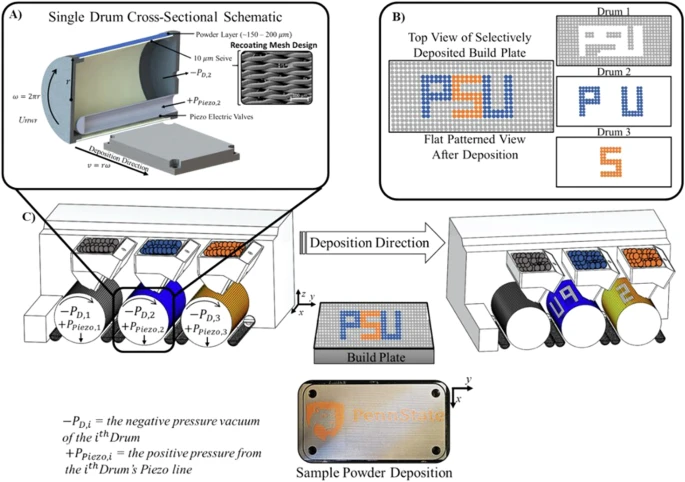
Tailored Properties and Complex Geometries
Multi-metal printing cracks open the toolbox for engineers by letting them blend alloys inside a single part, dialing in stiffness, toughness, wear life, or heat flow exactly where each is needed. Instead of relying on one monolithic composition, designers can now sculpt micron-scale zones that do very different jobs—yet grow together in one uninterrupted build.
💪 Variable Stiffness: Biomedical hardware often walks a tightrope between strength and flex. With multi-metal AM, a rigid Ti-6Al-4V skeleton can be intertwined with lattices of pure, more ductile titanium, mimicking the give-and-take of real bone and cutting stress-shielding almost to zero.
🛡️ Wear Resistance: Moving parts love built-in lubrication. Rather than press-fitting a brass bushing later, the printer can lay down a self-lubricating bronze sleeve during the build, right inside a steel housing—no joints, no glue, no future rattle.
🔥 Thermal Management: Hot spots beg for copper’s heat-soak, while the rest of the part still needs steel’s muscle. Multi-metal AM lets engineers snake pure-copper cooling channels through a stainless block or rocket nozzle throat, whisking heat away without sacrificing structural backbone. (2-a, 7-a)
🌐 Functionally Graded Materials: Thanks to SPD, an alloy can fade gradually into another instead of meeting at a hard line. That continuous gradient shrugs off corrosion on the surface while keeping high strength inside—a slick way to dodge the weak joints that haunt traditional bimetal builds.
Because these techniques liberate geometry from material limits, lightweight lattices such as gyroids or TPMS meshes can now be printed with each strut made of the alloy best suited to its load or thermal chore. One recent demonstration built a gyroid beam whose inner webs carried the muscle of stainless steel while the outer skin delivered bronze’s corrosion and heat perks—an open-cell heat-exchanger shape no mill or lathe could hope to carve.
Key Challenges in Multi-Metal Printing
Great promise brings fresh hurdles. Each alloy marches to its own thermal tune, so keeping them in step during a print is tricky.
• Material Compatibility: Dissimilar melting points and expansion rates can lock in residual stress or seed cracks where metals meet. A copper–steel pair, for example, demands laser power and scan speed that treat both fairly.
• Interfacial Defects: If powder placement or laser focus slips, pores and micro-cracks bloom along the boundary. Build orientation alone can swing porosity patterns from benign to catastrophic.
• Process Control: The recoater has to sprinkle each powder cleanly—zero cross-talk—while the machine flips laser parameters on the fly. Any blur in that dance can wreck the part.
• Quality Inspection: Single-alloy pieces are tough enough to check; mixed-metal parts need micro-CT and DIC to chase flaws hiding deep inside, adding cost and lead time.
• Post-Processing: Heat-treating one alloy might over-age its neighbor, so finishing steps must juggle multiple recipes—or invent new ones.
🔍 Mitigations are racing forward: real-time melt-pool cameras, thermal sensors, and AI-guided control loops tweak laser power layer-by-layer. Digital twins predict trouble spots before a single layer is laid, while machine-learning models have already cut parameter-tuning time in half for tricky alloy pairs such as stainless steel and Inconel. Together, these tools are pushing multi-metal AM from daring lab demo to dependable shop-floor reality.
Advantages and Real-World Applications
Multi-metal 3D printing has thrown open the innovation gates, letting engineers bake several working features into one seamless part—no bolts, no bonding, just one clean build. Here’s why that’s a game-changer:
✅ Design Freedom: Graded lattices, serpentine fluid channels, and what used to be six-piece sub-assemblies now roll off the printer as a single component. Prototype heat exchangers, for example, weave high-conductivity passages straight through load-bearing ribs without a hint of post-machining.
✅ Material Efficiency: Precious or scarce alloys land only where the physics demand them. Picture a turbine vane that spends its pricey high-temperature alloy exclusively at the fiery leading edge while relying on a common workhorse alloy elsewhere—saving metal, money, and migraines.
✅ Enhanced Performance: Printing dissimilar metals in situ wipes out manual joining, boosting reliability overnight. Studies and factory floors alike showcase multi-metal heat exchangers, rocket and jet-engine hardware, orthopedic implants, and custom tooling. One Penn State gyroid, for instance, targets solid-state energy storage while doubling as a biodegradable implant—its porous bronze bone-growth surface fused to a steel core for strength.
✅ Vibration Damping: From aerospace trusses to Paris–Roubaix-level bike forks, certain zones must flex while neighbors stay rock-solid. Multi-metal AM prints those stiff braces and compliant hinges in a single pass (Aerosint notes this exact trick). Imagine a bike frame that keeps a rigid joint yet slips a softer alloy into flex-critical stays, turning road chatter into a whisper and rider fatigue into yesterday’s news.
✅ Thermal Management: Molds, brake calipers, and even rotor hats crave both brute strength and rapid heat bleed. With multi-metal printing, copper cooling veins snake through a tough tool-steel block, scrubbing away heat faster than any drilled passage could dream. Likewise, a caliper body can emerge in a high-strength alloy while its brake face shines in copper or bronze for scorching-fast thermal transfer.
✅ Wear & Corrosion Resistance: Mining bits and injection-mold inserts live hard, die young—unless their surface layer is printed in a wear titan like cobalt-chrome while the core stays a cheaper, tougher steel. No brazed inserts, no weak seams, just one rugged, ready-for-battle part.
Real-world proof is piling up. Fraunhofer IGCV engineers fused nickel superalloy with copper to create rocket-thruster segments that shrug off blistering heat yet cool themselves efficiently (see their Ni/Cu nozzles). Renishaw and Siemens followed suit with stainless-steel brackets wearing cobalt-chrome armor, while Empire Cycles printed the first metal bike frame by blending aluminum alloys for sweet spot compliance and strength. No compromise—each metal delivers its superpower minus a single weld.
Bottom line? Tailored-property metal printing kills the old trade-off mindset. Where designers once settled for a “good-enough” alloy, multi-metal AM serves exactly what every zone craves—nothing more, nothing less. Aerospace, automotive, energy, and medical outfits are already lining up, hungry for lighter, smarter, multifunctional hardware. When you can print a part that’s leaner overall, tougher in the hotspots, and wired with extra functions, why on earth would you settle for anything less?
Future Outlook in Advanced Metal AM
The road ahead for Multi-Metal 3D Printing looks nothing short of exhilarating—change is arriving quickly and all at once. Researchers and manufacturers alike are sprinting to unlock the next wave of capabilities, and the hottest topics on everyone’s whiteboard include:
🔹 More Materials, Multi-Phase Printing
Early successes mostly combined two metals in one build, yet the horizon clearly shows three (or more) alloys mingling layer-by-layer. Aerosint’s SPD system can already sprinkle three different powders across a single slice at micron-scale precision, while next-gen binder-jet platforms hint at depositing varied binders first—then back-filling each zone with a tailor-made alloy.
🔹 Software + AI Integration
Design tools are catching up fast. CAD suites such as nTopology now let engineers paint distinct materials onto individual lattice regions right inside the model. Meanwhile, AI/ML engines—think the xT SAAM project—crunch mountains of sensor data to learn which laser power, hatch spacing, or layer time suits every unique metal pairing. Result? The once-painful R-and-D loop shrinks from months to mere days.
🔹 Production-Scale Machines
Dedicated multi-metal LPBF rigs are no longer science-fair prototypes. Beyond Aerosint’s retrofits, heavyweights like 3D Systems and TRUMPF are betting big, building factory-ready printers that can earn aerospace and medical stamps of approval within just a few years.
🔹 In-Situ Quality & Certification
Critical parts demand bulletproof QA. Teams are wiring printers with pyrometers, high-speed cameras, and ML-powered dashboards to flag flaws the instant they appear. When these watchdogs mature, multi-metal builds will march through the same ironclad certification gauntlet that single-alloy AM already conquers.
🔹 Industrial Adoption
As the kinks straighten out, industry will chase true “killer apps.” Picture turbine blades with integral coatings, graded bone implants that fade seamlessly into living tissue, or tooling that combines stiffness with self-cooling copper arteries. From Penn State to Fraunhofer and countless private R&D cells across Europe and Asia, the rallying cry is the same: scale the lab marvels into shop-floor reality.
Bottom line? Multi-Metal 3D Printing supercharges all the classic AM promises—wild geometry, digital on-demand builds—by adding a brand-new lever: precise, voxel-level material control. Yes, hurdles in process stability and QA remain, yet strides made in 2024–2025 prove the tech is already paying its way in select niches. Expect deposition speeds to climb, algorithms to sharpen, and alloy mashups we haven’t even imagined. The reward will be metal parts that land lighter, flex harder where they must, and juggle multiple functions inside a single, spotless print. 🚀
References
Penn State Univ. (2025). Beyond welding: Researchers 3D print two metals in single complex structure. (PSU news release on multi-metal 3D printing)psu.edu.
Griffis, J. C., Shahed, K., Meinert, K., Yilmaz, B., Lear, M., & Manogharan, G. (2025). Multi-material laser powder bed fusion: effects of build orientation on defects, material structure and mechanical properties. npj Advanced Manufacturing, 2, Article 5. (Study on multi-material LPBF)nature.com.
Metal AM. (2025). Penn State researchers achieve breakthrough in multi-metal additive manufacturing. (Industry news piece)metal-am.com.
Aerosint. (2021). Use cases for multi-metal 3D printing. (Industry blog post on tailored properties)aerosint.com.
Seidel, C. (2022). Multi-material metal parts by Powder Bed Fusion: New application opportunities. Metal Additive Manufacturing, 8(2), 15–18. (Review of multi-material PBF)metal-am.com.
Bietags, M. (2022). Multi-Material LPBF: 2x Faster with Machine Learning. Exponential Technologies. (Blog on ML-enhanced multi-material LPBF)x-t.ai.